Sie befinden sich hier
Inhalt
A. Bongers, C. Rehm, L. R. Schad
Introduction
Oxygen concentration plays a central role for the sensitivity of tumors to radiation- and chemotherapy. Hypoxic tumors show significantly higher resistance to these therapies and tend to be more malignant than tumors with normal oxygen content [1]. Hence, to increase blood oxygen saturation in tumors, in some settings radiation therapy patients are supplied with gases of high oxygen content (100 % O2 and Carbogen (95 % O2 / 5 % CO2)).
Monitoring of oxygen saturation and oxygen delivery in tissues is an important issue for tumor prognosis. A promising tool to noninvasively measure oxygen delivery into tisues can be MR-imaging utilizing the BOLD Effect [2]. This technique exploits the sensitivity of T2* measurements to field inhomogenieties induced by deoxygenated Hemoglobin.
To evaluate the possibility to measure the increase of oxygen delivery when patients inhale high oxygen content gases, this study examines the modulation of the BOLD-signal by two inhalation gases (100 % O2 and Carbogen (95 % O2 / 5 % CO2)). As a prerequisit for the use of this technique in patients the sensitivity of FLASH and EPI Imaging Sequences to Oxygen / Carbogen Inhalation was investigated in 5 volunteers.
Materials and methods
For each volunteer 4 measurements were performed. A T2* weighted flow-rephased FLASH- and a blipped GE-EPI- Sequence were investigated under inhalation of Carbogen (95 % O2 / 5 % CO2) and pure oxygen, respectively.
The measurements were performed on a Siemens Vision 1,5T hole body scanner, with the following sequence parameters:
EPI:
TE = 60ms, TR = 3s, Flipwinkel a = 90°, Aufnahmezeit 110 ms /Schicht, Matrix 128x128, FOV=220 mm,
Schichtdicke = 5mm
FLASH:
TE = 48ms, TR = 76ms, Flipwinkel a =30°, Bandbreite in RO-Richtung: 28 Hz / Pixel, Matrix 108x128, FOV = 220mm, Schichtdicke = 5mm
While supplying the volunteer with the gas mixtures (10 l / min) via an inhalation mask, data acquisition was performed in a continous series with time resolution of 3s for EPI and 8s for FLASH.
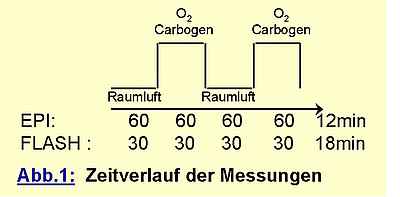
The data were postprocessed by correlating the measured signal pixel by pixel with the boxcar function depicted above. The resulting maps were thresholded at a significance level of p=0,001. For pixels with significant signal enhancement from gas inhalation the signal increase relative to the baseline was calculated and encoded in a percent scale colortable. As a measure of relative BOLD signal increase the average over all significant pixels was calculated where large vessels (indicated by signal changes larger than 20%) were excluded.
Results and discussion
Fig. 2 shows a typical case comparing the realtive signal enhancements of EPI and FLASH. The signal for Carbogen breathing with EPI data acquisition (Fig. 2 left) correlates strongly with the location of gray matter, while white matter does not show any significant signal enhancement. This finding reflects the higher capillary density and oxygen consumption in gray matter.
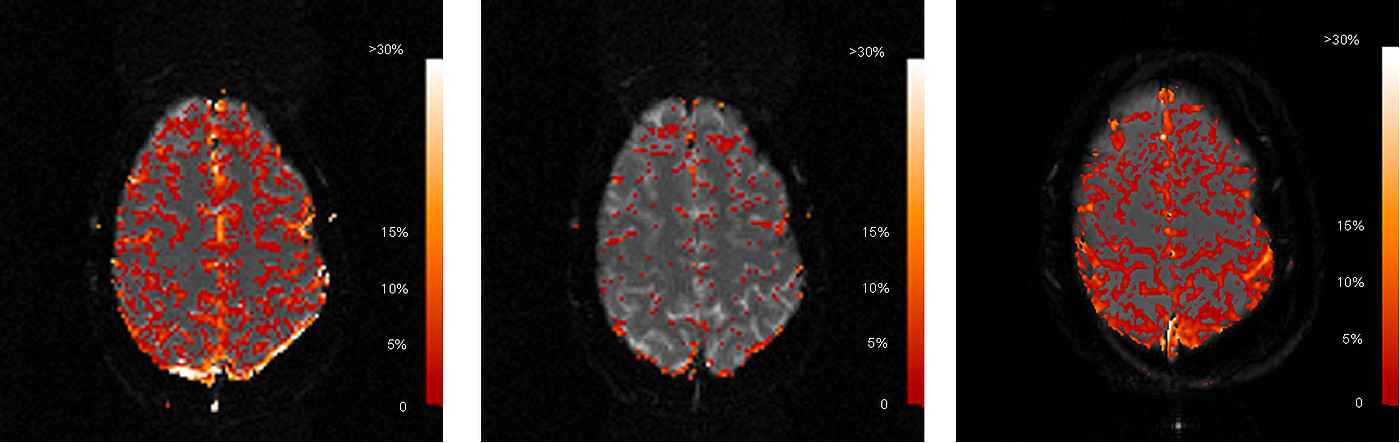
Generally, FLASH acquisitions schowed more stable correlation to gas inhalation than EPI. This was especially crucial for the calculation of correlation maps from pure oxygen. While EPI showed very poor correlation (Fig. 2 middle) with pure oxygen inhalation of the volunteers, it was possible to reproducibly image gray matter with the FLASH technique for this modality (Fig. 2 right). The increased signal stability for FLASH is a result of higher SNR due to the lower bandwidth in RO-direction.
Average signal enhancement in the parenchyma was calculated in the order of 5 % for both gases with interindividual variations of 2 %. Although average T2* increase for Carbogen-compared to Oxygen breathing was consistently 0,5-1% higher, differences were not significant due to the high standard deviation of pixels within the parenchyma. Large vessels showed relative signal enhancements of 15-20 % for Oxygen and 25 % for Carbogen.
References
[1] Molls, M., P. Stadler, et al. (1998). “Relevance of oxygen in radiation oncology. Mechanisms of action, correlation to low hemoglobin levels.” Strahlenther Onkol 4: 13-6
[2] Robinson, S. P., D. R. Collingridge, et al. (1999). “Tumour response to hypercapnia and hyperoxia monitored by FLOOD magnetic resonance imaging.” NMR Biomed 12(2): 98-106