Sie befinden sich hier
Inhalt
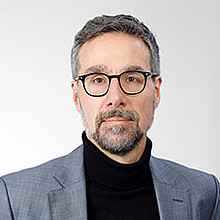
Department of Neurology Medical Director
Professor of Neurology
KKE Neuroimmunology and Brain Tumor Immunology, DKFZ Heidelberg
Neurology - Neuroinflammation
The central nervous system (CNS) is regarded as an immune-privileged organ in which immune responses are strictly controlled by intensive exchange with the peripheral immune system despite the blood-brain barrier. Despite this strict control, autoimmune diseases can occur in the CNS. A paradigmatic disease is multiple sclerosis (MS). In contrast, intrinsic brain tumors of the CNS, especially gliomas, often lead to immunosuppression through active processes.
Our Clinical Cooperation Unit Neuroimmunology and Brain Tumor Immunology – a joint research division of the Medical Faculty Mannheim, Heidelberg University and the German Cancer Research Center aims at understanding and therapeutically exploiting the control of CNS autoimmunity and at developing novel immunotherapeutic approaches for brain tumors. To this end, we are developing innovative animal models and working with patient material. The overall aim of these works is the rapid translation of the findings into clinical studies and to implement an iterative cycle of target discovery and treatment development (Figure 1).
These efforts are embedded in several collaborative research efforts such as the Collaborative Research Centers SFB1389 and SFB1366, the Hertie Network of Excellence in Clinical Neuroscience, the Research Training Groups RTG2099, and RTG2727 the Helmholtz Future Topic Immunology and Inflammation, the German Cancer Consortium and the Helmholtz Institute for Translational Oncology.
Metabolic control of autoimmunity and antitumor immunity
In recent years, we have identified key metabolic events that regulate immune responses in the context of multiple sclerosis and brain tumors These findings have opened new perspectives on the development of immunotherapeutic drugs.
The finding that tryptophan metabolites (kynurenines) generated by the activity of tryptophan-2,3-dioxygenase (TDO) promote tumor growth by activating the aryl hydrocarbon receptor (AHR) and suppresses neuroinflammation – also through modulating the gut microbiome - raises a number of further questions that we are trying to answer with the help of new MS animal models and tumor models (Figure 2 and 3). A key goal is to identify drugs that interfere with tryptophan catabolism as a possible treatment for MS, malignant gliomas and other types of cancer. An AHR inhibitor developed within the DKFZ-Bayer Immunooncology Alliance is currently undergoing Phase 1 clinical testing.
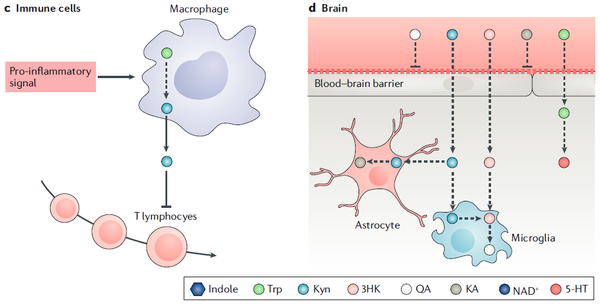
Certain types of brain tumors harbor oncogenic mutations in the gene for isocitrate dehydrogenase type 1 (IDH1) resulting in the accumulation of 2-hydroxyglutarate (2-HG), an oncometabolite that drives progression of brain tumors. The discovery that 2-HG is taken up by tumor-infiltrating immune cells and paralyzes their function opens new therapeutic options for 2-HG inhibitors in the context of immunotherapy for brain tumors (Figure 4).
Plasticity and function of myeloid cells in the CNS
Tumor-infiltrating myeloid cells play a key role in the immune response against brain tumors. Although these cells initiate and amplify immune responses against the tumor, cancer cells use tumor-infiltrating myeloid cells to promote tumor growth by angiogenesis and immunosuppression. A high density of these tumor infiltrating myeloid cells is associated with a poor prognosis. Although some of the crucial molecular processes in tumor infiltrating myeloid cells are known, such as the expression of checkpoint inhibitors on macrophages or the activation of certain metabolic processes (TDO/IDO activation), there is still a lack of concrete targets for a targeted therapy against the tumor promoting effect of the myeloid cells (Figure 5 and 6).
Therefore, the aim of our work is to identify new targets for the modulation of immunity in the CNS with regard to autoimmunity and tumor immunity in the CNS as well as in the context of neurodegenerative and psychiatric diseases. New imaging parameters should help to assess the dynamic changes of this immune compartment in animal models and in patients.
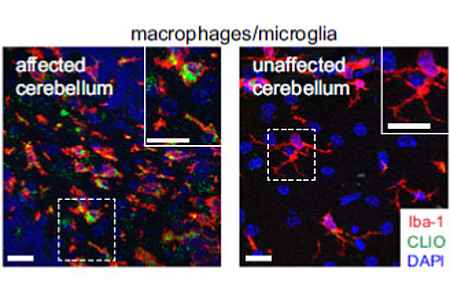
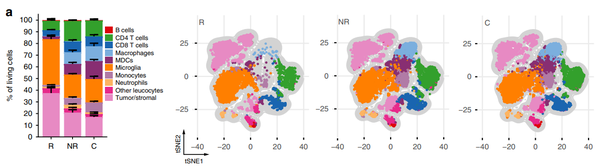
Antigen-specific T cell immunity
A particular focus in recent years has been on the identification of mutation-specific T cell responses to brain tumors and its therapeutic exploitation for tumor vaccines. Based on the preclinical characterization of an antigen-specific brain tumor vaccine according to human brain tumor tissue and humanized mouse modes we have demonstrated safety and immunogenicity of this vaccine in a publicly funded multicenter first-in-man phase 1 clinical trial supported by the Neurooncology Working Group of the German Cancer Society. To understand the complex immunological response to treatment a multicenter phase 1 window-of-opportunity trial is currently being conducted. Platforms to identify and functionally test tumor-reactive T cells from brain tumor tissue have recently been established and validated in a clinical trial (Figure 7 and 8).
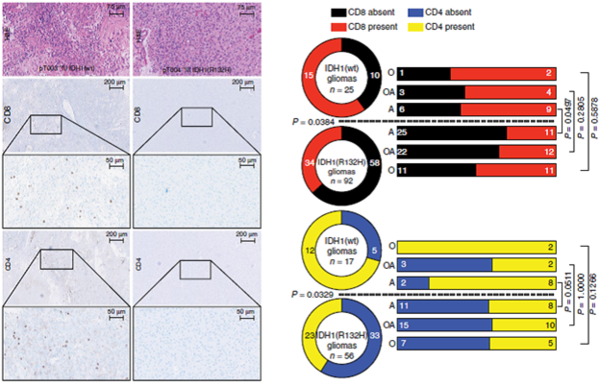
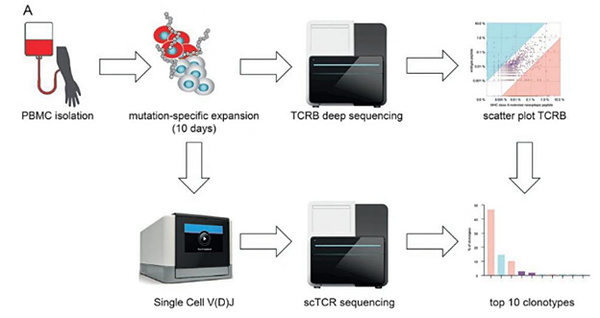
Noteworthy accomplishments
EU: ERC Advanced Grant “Characterizing and Harnessing Tumor-reactive T Cells in the Brain“ (CENTRIC-BRAIN); Duration: 2024 – 2029
Selected national and international joint research projects
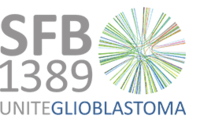
DFG: CRC 1389 / 2nd funding phase “Understanding and Targeting Resistance in Glioblastoma” (UNITE Glioblastoma), Co-speaker: Michael Platten, Project duration: 07/2023 – 06/2027
- Subproject B01: Mechanisms of response and resistance to glioma-specific T-cells (Theresa Bunse/Michael Platten)
- Subproject B03: Targeting immunosuppressive programs in isocitrate dehydrogenase mutant gliomas (Stephan Pusch/Lukas Bunse)
- Subproject B06N: Visualization and characterization of immune responses in H3K27M mutant gliomas (Michael Breckwoldt/Katharina Sahm)
DFG: CRC 1389 / 1st funding phase "Understanding and Targeting Resistance in Glioblastoma” (UNITE Glioblastoma), Co-speaker: Michael Platten, Project duration: 07/2019 – 06/2023
- Subproject B01: Mechanisms of response and resistance to checkpoint blockade in gliomas (Theresa Bunse/Michael Platten)
- Subproject B03: Targeting Immunosuppressive progams in isocitrate dehydrogenase mutant gliomas (Stefan Pusch/Lukas Bunse)
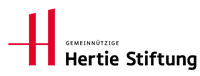
Hertie foundation: Hertie Network of Excellence in Clinical Neuroscience
- Hertie Fellow 2023: Dr. Dr. Lukas Bunse „Development of genetically modified T cells equipped with specific immune receptors to effectively target primary brain tumors“
- Hertie Fellow 2020: Dr. Katharina Sahm, “Improvement of targeted vaccines for the treatment of malignant brain tumors”

DFG: SPP 2395 “Local and peripheral drivers of microglial diversity and function”; Duration: 01/2023 – 12/2025
- Subproject: Microglial immunosurveillance of oncogenic IDH – (CONVINCE-IDH) (Michael Platten/Lukas Bunse)
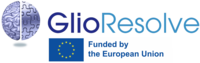
EU: Training the next-generation of European GLIOblastoma (translational) researchers, to RESOLVE precision targeting of the brain tumour microenvironment (GlioResolve); Duration: 09/2022 – 08/2026
- PhD project: Targetability of IDHwt GBM TME subtype – specific T cell and GBM associated endothelial cell interactome
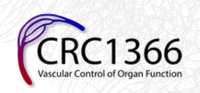
DFG: CRC 1366 / 2nd funding phase “Vascular Control of Organ Function”, Project duration: 01/2023 – 12/2026
- Subproject C01: Vascular control of tumor immunity in the central nervous system (Katharina Sahm/Michael Platten)

German Cancer Aid: A MultIceNTer PhasE I RNA VaCcine Trial to Exploit NeoePitope-Specific T Cells for the Treatment of H3K27M-Mutated Gliomas (INTERCEPT H3); Duration: since 2022
German Cancer Aid: Regulation of tumor immunity through the integrated stress response (ISR) in myeloid cells; Duration: 07/2020 – 06/2023
DFG: Research Training Group RTG 2727 “Innate Immune Checkpoints in Cancer and Tissue Damage” (InCheck); Duration: 01/2022 – 06/2022
- Subproject B.1.1: Modulating innate checkpoints for brain tumor immunotherapy (together with Katharina Sahm).
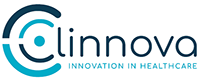
MWK: Cross-border digital health initiative; Duration: since 2021
- Subproject: Use case Multiple Sclerosis (project lead: Prof. Dr. Lucas Schirmer)
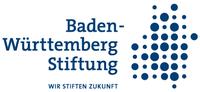
Baden-Württemberg foundation: REsolvInG ImmuNITy to targEt Brain Tumors (RE-IGNITE) (Joint project with Maximilian Häussler); Duration: 01/2020 – 11/2023
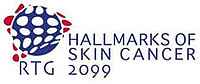
DFG: Research Training Group RTG 2099 “Hallmarks of Skin Cancer: Tumor-Micromilieu and Melanom-Immunology”; Duration: 10/2019 – 03/2024
- Subproject “Response and Resistance to Checkpoint Blockade in Melanoma Brain Metastases”.
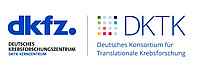
DKTK, DKFZ: AMPLIFYing NEOepitope-specific VACcine Responses in progressive diffuse gliomas (AMPLIFY-NEOVAC); Duration: since 2018
- ClinicalTrials.gov Identifier: NCT03893903
- Clinical trial protocol: AMPLIFY-NEOVAC: a randomized, 3-arm multicenter phase I trial to assess safety, tolerability and immunogenicity of IDH1-vac combined with an immune checkpoint inhibitor targeting programmed death-ligand 1 in isocitrate dehydrogenase 1 mutant gliomas. Bunse et al.; Neurol Res Pract. 2022. 4(1):20. doi: 10.1186/s42466-022-00184-x
DKTK, DKFZ: Coordinator of AMplify Immunological Insights by Glioma Organoids (AMI2GO); Duration: 09 /2021 – 08 /2024
DKTK, DKFZ: Immune response CAR-NK immunotherapy in glioblastoma (CAR2BRAIN); Duration: 01/2020 – 12/2022

Dr. Rolf M. Schwiete foundation: T cell receptor-transgenic T cell therapy for brain tumor patients; Duration: 09/2017 – 12/2021
Legend: DFG = German Research Foundation, BMBF = Federal Ministry of Education and Research, EU = European Union, MWK = Ministry of Science, Research and the Arts, CRC = Collaborative Research Center, DKTK = German Cancer Consortium, DKFZ = German Cancer Research Center, SPP = priority programme
Selected publications
- H3K27M-targeted vaccine in adults with diffuse midline glioma.
Grassl N, Poschke I, Lindner K, Bunse L, Mildenberger I, Boschert T, Jähne K, Green EW, Hülsmeyer I, Jünger S, Kessler T, Suwala AK, Eisele P, Breckwoldt MO, Vajkoczy P, Grauer OM, Herrlinger U, Tonn JC, Denk M, Sahm F, Bendszus M, von Deimling A, Winkler F, Wick W, Platten M*, Sahm K* (2023). Nat Med. 29(10):2586-2592. *equal contribution doi: 10.1038/s41591-023-02555- - MHC class II-restricted antigen presentation is required to prevent dysfunction of cytotoxic T cells by blood-borne myeloids in brain tumors.
Kilian M, Sheinin R, Tan CL, Friedrich M, Krämer C, Kaminitz A, Sanghvi K, Lindner K, Chih YC, Cichon F, Richter B, Jung S, Jähne K, Ratliff M, Prins RM, Etminan N, von Deimling A, Wick W, Madi A, Bunse L, Platten M (2023). Cancer Cell 41(2):235-251.e9. doi:10.1016/j.ccell.2022.12.007 - T cell-independent eradication of experimental glioma by intravenous TLR7/8-agonist-loaded nanoparticles.
Turco V, Pfleiderer K, Hunger J, Horvat NK, Karimian-Jazi K, Schregel K, Fischer M, Brugnara G, Jähne K, Sturm V, Streibel Y, Nguyen D, Altamura S, Agardy DA, Soni SS, Alsasa A, Bunse T, Schlesner M, Muckenthaler MU, Weissleder R, Wick W, Heiland S, Vollmuth P, Bendszus M, Rodell CB, Breckwoldt MO, Platten M (2023). Nat Commun 14(1):771. doi:10.1038/s41467-023-36321-6 - A vaccine targeting mutant IDH1 in newly diagnosed glioma.
Platten M, Bunse l, Wick A, Bunse T, Le Cornet L, Harting I, Sahm F, Sanghvi K, Tan CL, Poschke I, Green E, Justesen S, Behrens G, Breckwoldt M, Freitag A, Rother LM, Schmitt A, Schnell O, Hense J, Misch M, Krex D, Stevanovic S, Tabatabai G, Steinbach JP, Bendszus M, von Deimling A, Schmitt M, Wick W (2021). Nature 592:463-468. doi:10.1038/s41586-021-03363-z - Tryptophan metabolism drives dynamic immunosuppressive myeloid states in IDH-mutant gliomas.
Friedrich M, Sankowski R, Bunse L, Kilian M, Green E, Ramallo Guevara C, Pusch S, Poschet G, Sanghvi K, Hahn M, Bunse T, Münch P, Gegner H, Sonner J, von Landenberg A, Cichon F, Aslan K, Trobisch T, Schirmer L, Abu-Sammour D, Kessler T, Ratliff M, Schrimpf D, Sahm F, Hopf C, Heiland D, Schnell O, Beck J, Böttcher C, Fernandez-Zapata C, Priller J, Heiland S, Gutcher I, Quintana F, von Deimling A, Wick W, Prinz M and Platten M. (2021). Nature Cancer. doi:10.1038/s43018-021-00201-z - Heterogeneity of response to immune checkpoint blockade in hypermutated experimental gliomas.
K Aslan, V Turco, J Blobner, J K Sonner, AR Liuzzi, NG Nunez, D De Feo, P Kickingereder, M Fischer, E Green, A Sadik, M Friedrich, K Sanghvi, M Kilian, F Cichon, L Wolf, K Jahne, A von Landenberg, L Bunse, F Sahm, D Schrimpf, J Meyer, A Alexander, G Brugnara, R Roth, K Pfleiderer, B Niesler, A von Deimling, C Opitz, M O Breckwoldt, S Heiland, M Bendszus, W Wick, B Becher and M Platten (2020). Nat Commun 11(1):931. doi:10.1038/s41467-020-14642-0 - Dietary tryptophan links encephalitogenicity of autoreactive T cells with gut microbial ecology.
Sonner JK*, Keil M*, Falk-Paulsen M*, Mishra N, Rehman A, Kramer M, Deumelandt K, Röwe J, Saghvi K, Wolf L, von Landenberg A, Wolff H, Bharti R, Oezen I, Lanz TV, Wanke F, Tang Y, Brandao I, Mohapatra S, Epping L, Grill A, Röth R, Niesler B, Meuth SG, Opitz CA, Okun JG, Reinhardt C, Kurschuss F, Wick W, Bode HB, Rosenstiel P*, Platten M* (2019). Nat Commun Oct 25;10(1):4877. *equal contribution doi:10.1038/s41467-019-12776-4 - Suppression of antitumor T cell immunity by the oncometabolite R-2-hydroxyglutarate.
Bunse L*, Pusch S*, Bunse T*, Sahm F, Sanghvi K, Friedrich M, Alansary D, Sonner JK, Green E, Deumelandt K, Kilian M, Neftel C, Uhlig S, Kessler T, von Landenberg A, Berghoff AS, Marsh K, Steadman M, Zhu D, Nicolay B, Wiestler B, Breckwoldt MO, Al-Ali R, Karcher-Bausch S, Bozza M, Oezen I, Kramer M, Meyer J, Habel A, Poschet G, Weller M, Preusser M, Nadji-Ohl M, Thon N, Burger M, Harter P, Ratliff M, Harbottle R, Benner A, Schrimpf D, Okun J, Herold-Mende CM, Turcan S, Kaulfuss S, Hess-Stumpp H, Bieback K, Cahill DP, Plate KH, Hänggi D, Dorsch M, Suva M, Niemeyer BA, von Deimling A, Wick W, Platten M (2018). Nat Med 24:1192-1203. *equal contribution doi:10.1038/s41591-018-0095-6 - A vaccine targeting mutant IDH1 induces antitumor immunity.
Schumacher T*, Bunse L*, Pusch S, Sahm F, Wiestler B, Quandt J, Menn O, Osswald M, Oezen I, Ott M, Keil M, Balss J, Rauschenbach K, Grabowska AK, Vogler I, Diekmann J, Trautwein N, Eichmüller S, Okun J, Stefanovic S, Riemer AB, Sahin U, Friese M, Beckhove P, von Deimling A, Wick W, Platten M (2014). Nature 512:324-327. *equal contribution doi:10.1038/nature13387 - An endogenous tumour-promoting ligand of the human aryl hydrocarbon receptor.
Opitz CA, Litzenburger UM, Sahm F, Ott M, Tritschler I, Trump S, Schumacher T, Jestaedt L, Schrenk D, Weller M, Jugold M, Guillemin GJ, Miller CL, Lutz C, Radlwimmer B, Lehmann I, von Deimling A, Wick W, Platten M (2011). Nature 478:197-203. doi:10.1038/nature1049
Photo Credits: #1 FGV-Zentrum, Med. Fakultät Mannheim
Kontextspalte
Contact
Medical Faculty Mannheim
Heidelberg University
Theodor-Kutzer-Ufer 1-3
68167 Mannheim
Phone +49 621 383-2885
michael.platten@ medma.uni-heidelberg.de