Sie befinden sich hier
Inhalt
In the first funding period, the research program of the RTG aimed at elucidating the cellular/molecular pathways that lead to skin cancer cell dissemination (Research Area A), and at analyzing the mechanisms of primary skin cancer resistance to cell death and tumor immunity (Research Area B). In a cross-sectional approach, all projects aimed at identifying novel targets against the hallmarks of skin cancer they studied.
Although the Hallmarks of Cancer concept has not been revised since the start of the RTG, we hold that it is still a valid guideline to define research directions in the complex and evolving field of melanoma biology and immunity. Due to the extraordinary achievements in melanoma immunotherapy, we now focus solely on malignant melanoma in the second funding period. In this regard, we expand research into melanoma immunity and immunotherapy at the expense of mechanisms of cell death while keeping the successful focus on the melanoma tumor microenvironment also in the second funding period. Therefore, the RTG continues to analyze hallmarks of skin cancer and focuses on intercommunicative hallmarks of cancer within the tumor microenvironment such as interactive signaling and vascular control of metastasis (Research Area A) and on innate and adaptive mechanisms of tumor immune control (Research Area B) in malignant melanoma. Within the RTG, the projects are organized in project packages to support intensive collaboration. The projects share a broad spectrum of modern molecular, genetic, biochemical, cell biological, and morphometric techniques. The RTG benefits from this exchange of expertise, both in terms of competitive research and of educational needs.
Research Area A – Tumor Microenvironment
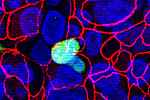
The interactions between the tumor microenvironment and malignant melanoma on a local and systemic level are the major focus of Research Area A. Projects 1-4 investigate inside out signaling and outside in signaling at the primary site of melanoma progression as well as the effects that the primary tumor exerts on cancer cell dissemination to distant sites. Projects 5-8 study vascular mechanisms of metastasis to the regional lymph nodes, the brain and the liver taking into account that the primary may already have altered the (pre)metastatic niche in the recipient organ. Although completion lymph node dissection in sentinel lymph node-positive melanoma patients does not improve overall survival, the prognostic value of sentinel lymph node positivity and also of non-sentinel lymph node positivity is paramount.
Project Package A1: Interactive Signaling in Melanoma Growth and Dissemination
P1: Dissecting the role of Wnt signaling in melanoma
(PI: M. Boutros; London PI: B. Baum)
P2: Communication between tumor cells and fibroblasts in the tumor microenvironment during melanoma progression
(PI: P. Angel; London PI: E. Sahai)
P3: Role of systemic osteopontin and senescence in melanoma metastasis and therapy resistance
(PI: J. Sleeman; London PI: Caroline Hill)
P4: The role of N-Fragment of Angiopoietin-like 4 during malignant melanoma metastasis formation
(PIs: M. Felcht, H. Augustin; London PI: K. Hodivala-Dilke)
Project Package A2: Vascular Control of Melanoma Metastasis
P5: The lymph node vascular niche in melanoma metastasis
(PIs: P. Reiners-Koch, S. Goerdt; London PI: T. Ng)
P6: Role of YAP/TAZ in the endothelium of melanoma brain metastasis
(PI: C. Ruiz de Almodóvar Egea; London PI: E. Sahai)
P7: Mechanisms of T cell homing and function in melanoma brain metastases
(PIs: M. Karreman, F. Winkler; London PI: E. Sahai)
P8: Pre-metastatic and immunologic conditioning of the hepatic vascular niche during melanoma progression
(PIs: S. Wohlfeil, C. Géraud; London PI: K. Hodivala-Dilke)
Research Area B – Tumor Immunity / Immunotherapy
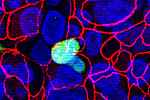
The major focus of Research Area B is to scrutinize melanoma immunity in order to overcome innate immunity-mediated immunosuppression (projects 9-11) and in order to improve the adaptive T-cell armamentarium against malignant melanoma (projects 12-15).
Project Package B1: Innate Mechanisms of Melanoma Immunity
P9: Defining innate immune checkpoints controlling Natural Killer cell recognition of melanoma cells
(PI: A. Cerwenka; London PI: A. Hayday; Paris PI: L. Zitvogel)
P10: Role of the glucocorticoid-induced P2Y12/P2Y14+ G-protein coupled receptors for TAM in melanoma
(PI: A. Schmieder; London PI: T. Lawrence; Paris PI: C. Robert)
P11: Stimulation of myeloid cell-mediated immunosuppression via TLR signaling and its targeting in melanoma
(PI: V. Umansky; London PI: S. Quezada; Paris PIs: F. Mami-Chouaib, A. Marabelle)
Project Package B2: Checkpoint Inhibition and Adaptive Melanoma Immunotherapy
P12: The Role of the Immune Checkpoints LAG-3 and TIM-3 for Immunotherapy of Melanoma
(PI: J. Hassel; London PI: J. Larkin; Paris PIs: A. Eggermont, A. Marabelle)
P13: Differentiation of induced pluripotent stem cells into tumor antigen-specific T cells for melanoma immunotherapy
(PIs: M. Gaiser, J. Utikal; London PI: P. Guermonprez; Paris PIs: L. Zitvogel, A. Marabelle)
P14: Response and Resistance to Checkpoint Blockade in melanoma brain metastases
(PIs: T. Bunse, M. Platten; London PI: S. Quezada; Paris PI: L. Zitvogel)
P15: Regulation of anti-tumor immune responses by nutrient availability in melanoma
(PIs: G. Cui, V. Umansky; London PI: D. Anastasiou; Paris PI: S. Chouaib)
Background
Melanoma immunotherapy with anti-CTLA4 and anti-PD1 antibodies has revolutionized Dermato-Oncology changing paradigms not only for skin cancer therapy, but also for the treatment of solid cancers in general. Anti-PD1 immunotherapy is more effective compared to anti-CTLA4 immunotherapy in patients with advanced melanoma, however, the combination of anti-PD1 and anti-CTLA4 treatment is even better. Of course, this great advantage for melanoma patients comes at the expense of more adverse events. Melanoma immunotherapy with anti-PD1 and anti-CTLA4 antibodies is effective also in immune privileged organs including brain metastases, possibly due to peripheral expansion of homing-competent effector CD8+ T-cells, but further enhanced through modulation of the brain microvasculature allowing T-cell entry. In addition, anti-PD1 immunotherapy is now also offering effective adjuvant treatment in malignant melanoma for the benefit of the patients.
Further Background
Despite the dramatic improvements in the treatment of malignant melanoma, a larger proportion of patients do not yet show durable responses to immunotherapy. Three different lines of approaches to this problem are being investigated at the moment: improved patient selection, identification and targeting of resistance mechanisms, and combination treatment modalities. In desmoplastic melanoma, for example, high response rates to PD1 blockade may be achieved in advanced stages of the disease. In addition, HLA class I genotypes, the frequency of CD14+CD16-HLA-DRhi monocytes in the peripheral blood, PDL1 and CD137 expression on peripheral T-cell subpopulations, and β-catenin-mediated immune evasion, but also other patient parameters to be identified such as tumor mutational load may become valuable predictors of a response to checkpoint blockade immunotherapy upon development of personalized cancer immunotherapy approaches. Recently, actionable resistance mechanisms to cancer immunotherapy have been identified including mutations and epigenetic regulators of interferon-receptor signaling and antigen presentation.
Combination therapies, however, may hold the greatest promise in improving melanoma immunotherapy. Notably, the gut microbiota promote and shape the response of melanoma patients to both anti-CTLA4 and anti-PD1 immunotherapies. Quick translation of these findings may be possible through oral administration of the “good” bacteria or through fecal transplantation in combination with immune checkpoint inhibition in malignant melanoma. In addition, a whole plethora of molecular and cellular targets are investigated for the development of combination immunotherapies such as TNFα blockade, activation of GPER1 signaling, nanoparticle-anchored delivery of anti-CD137 antibodies or of IL-2 to the tumor microenvironment to circumvent lethal immunotoxicity, or enhancing T-cell homing. Last, but not least, immune cell metabolism is an evolving field with emerging targets for cancer immunotherapy. In malignant melanoma, promoting fatty acid catabolism improves the ability of CD8+ tumor infiltrating lymphocytes to slow tumor progression synergizing with immune checkpoint inhibition.
Immune checkpoint inhibition may also be combined with myeloid-targeted immunotherapies activating dendritic cells with anti-CD40 antibodies and inhibiting tumor-associated macrophages and myeloid-derived suppressor cells by anti-CSF1R antibodies or by anti-arginase1 immunization. Atypical scavenging chemokine receptors such as ACKR2 are also promising targets for myeloid checkpoint inhibition. In addition, the natural killer-dendritic cell axis defines patient responsiveness to anti-PD1 immunotherapy. Moreover, research into immune cell metabolism is also relevant in innate immunity. Braf-mutant melanoma cells, for example, produce high amounts of Wnt5a activating fatty acid oxidation in dendritic cells with downstream activation of IDO1 and generation of Treg cells. Other Wnt signaling components such as DKK2 may also be involved in tumor immune evasion. Therefore, nanoparticle-mediated trapping of Wnt family members in the tumor microenvironment may be combined with immune checkpoint inhibitors.
Tumor Microenvironment
Besides immunotherapy, Braf and MEK inhibition have added a second chapter to the triumphant development of melanoma therapy in recent years. Especially combination therapies with both Braf and MEK inhibitors together have benefitted patients regarding longer progression-free and overall survival. Similar to immunotherapies, combination therapies with Braf and MEK inhibitors are effective in brain metastases, as well as in adjuvant or neoadjuvant settings. However, 3-year overall survival strongly depends on the selection of patient subgroups ranging from approx. 60% in the most favorable subgroup to only 25% in the most unfavorable subgroup. To overcome resistance, novel targets are being identified by analysis of whole-genome landscapes of major melanoma subtypes, and comprise the ERK transcriptome, epigenetic regulators, as well as cancer cell metabolism.
Despite these developments, it becomes increasingly evident that targeting cell-autonomous cancer cell functions and signaling pathways may not suffice to fully eradicate malignant melanoma metastasis leaving ample room for identifying novel host-derived interactive targets. Metastasis is a multi-step process involving both cancer cells and the tumor microenvironment. Upregulation of core fucosylation by FUT8 is a prime example of how malignant melanoma drives metastasis by preparing the tumor microenvironment for matrix-guided cell migration. In primary melanoma, another precondition for metastasis is angiotropism followed by invasion of the tumor cells into tumor-associated micro-vessels. Primary tumors also interact with pre-metastatic sites preparing organ-specific pre-metastatic niches via soluble factors and circulating inflammatory cells. Circulating metastasis-initiating cells succumb to interactions with developing organ-specific metastatic niches, and secondary recirculating cancer cells interact back with the primary. Metastatic organotropism and metastatic disease progression are a result of this feed forward cycle of dynamic, reciprocal interactions of cancer cells with their diverse metastatic niches.
The regional lymph node (LN) usually is the first metastatic niche for malignant melanoma (MM). In line with this, sentinel-lymph node (SLN) status is the single most important prognostic factor in clinically LN-negative disease; the same applies to non-SLN status in SLN-positive disease. The median disease-free survival for patients with non-SLN-negative disease is considerably longer as compared to non-SLN-positive disease indicating an orderly progression from the SLN to the non-SLN compartment. In addition, lymphatic vessels are indispensable for metastatic spread and primary MM drives development of a pre-metastatic LN vascular niche through tumor-derived and stromal factors. Furthermore, recent evidence suggests that LN metastases show angiotropism similar to the primary, can invade local blood vessels, exit the node, and colonize distant organs. This indicates a hybrid route for melanoma cell dissemination involving both the lymphatic as well as the blood vascular systems. As neither SLN removal nor completion LN dissection increase MM-specific survival, it remains an open question whether lymphatic dissemination contributes to distant MM metastasis.
Underlining the importance of the diverse metastatic niches, genetic alterations driving metastatic spread are acquired outside the primary tumor in melanoma. However, there is ongoing discussion whether establishing metastasis in melanoma is a late-evolving trait in the primary with seeding the same clone to different recipient organ sites. A step further, induction of dormancy versus metastatic cancer cell death has been shown to depend on interferon-β-mediated activation of a metabolic checkpoint pathway. Notably, a genome-wide screen for novel host regulators of metastatic colonization identified the sphingosine-1-phosphate transporter spinster homolog2 (Spns2) as the most important molecule to reduce lung metastasis. Spns2 acts in a lymphatic endothelial-specific manner in the lung demonstrating the importance of the organ-specific vasculature within the metastatic niche.
In general, it is becoming increasingly obvious that the organ-specific vasculature acts as a dynamic interface that controls organ-specific functions during homeostasis, and disease including tumor growth and metastatic spread. To control organ function, vascular mural cells, especially endothelial cells, express angiocrine factors in an organ-specific manner which may act locally or systemically. This heterogeneity of vascular niches has been defined as organotypic angiodiversity. Organotypic angiodiversity and angiocrine functions have been analyzed paradigmatically in the liver, the brain, the bones, and in malignant tumors.
Notably, tumor angiogenesis and organotypic angiodiversity are mutually interconnected; tumor angiogenesis is blocked and metastasis is inhibited, for example, when the angiogenic growth factor Angpt2 is neutralized. Furthermore, angiocrine factors displayed by organ-specific endothelial cells control organotropism during metastasis. As a superordinate regulator of organotypic angiodiversity-mediated angiocrine factor expression, Notch signaling seems to be highly important, especially during metastatic processes. While pharmacological Notch inhibition caused an increase in liver metastasis, endothelial-specific Notch deletion caused a reduction in lung metastatic spread. With regard to therapy, this complex situation must be considered as Notch inhibition might be beneficial in lung metastasis, but detrimental regarding metastasis to the liver. In addition to angiocrine signaling, the β2-adrenergic receptor, Adbr2, has been identified as a novel checkpoint molecule in angio-metabolism that could potentially be targeted as an anti-cancer therapy. Besides endothelial cells, however, mural cells such as pericytes must not be neglected as they may also control metastasis. This notion holds also true for melanoma-associated fibroblasts (MAFs), a cell type not yet well characterized in malignant melanoma.